Molecular Modelling of Transport and Transfer Processes

The Helmholtz Institute Münster forms an interdisciplinary team for theoretical investigations in the area of electrolyte research. In cooperation with the WWU group of Physical Chemistry Dr. Diddo Diddens from HI MS involves molecular modelling of ion transport and electron transfer processes in energy materials in his habilitation project, especially with regard to batteries.

Ion Transport in Polymer Electrolytes
Polymer electrolytes are considered as promising alternatives to liquid electrolytes due to their mechanical integrity and low flammability, which is particularly important for lithium metal batteries. However, in many of these materials, the lithium transport is too low for an efficient use, necessitating further improvement. Via molecular dynamics (MD) simulations, Diddens aims to derive a fundamental understanding of molecular ion transport mechanisms and to assess their importance for macroscopic transport properties measured experimentally. These activities are carried out in cooperation with the theory group of Prof. Andreas Heuer.
On the basis of a mathematical model, it could be demonstrated that the use of low-molecular additives, which directly coordinate the lithium ions and decouple them from the slow polymer chains, can substantially enhance the lithium diffusion coefficient (1; see left image in figure 1). A similar decoupling of lithium ions from the coordinating polymer host can be observed for block copolymers doped with large amounts of lithium salt (2; right image in figure 1), which is reminiscent of polymer-in-salt electrolytes (3).
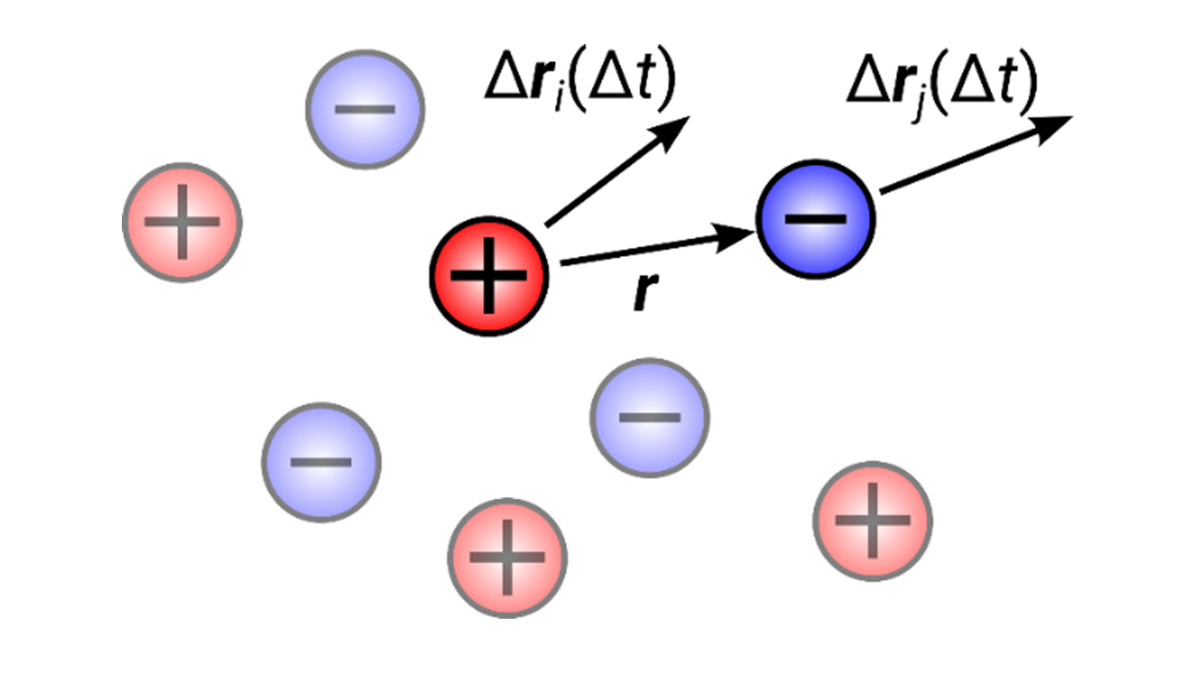
Understanding Dynamic Ion Correlations
For battery electrolytes the current carried by certain ion species, for example lithium ions, is a critical transport parameter, which is usually expressed by the product of two quantities: the overall conductivity and the transference number. While diffusion coefficients – characterizing the motion of single ions – can be separately measured for cations and anions (and solvent molecules), estimating the charge fraction carried by a given ion species is less trivial. This is due to the fact that ions move cooperatively, which is usually rationalized by the motion of ion pairs or clusters (see sketch in figure 2), such that the total transported charge deviates from the ions’ nominal charges. Diddens aims to understand these dynamical ion correlations in more detail via MD simulations (4).

Electrochemical Stability and Electrolyte Decomposition
Typical battery electrolytes are thermodynamically unstable against both metallic lithium and lithium-intercalated graphite or silicon anodes, however, continuous electrolyte decomposition can be prevented if a passivation layer, the so-called Solid Electrolyte Interphase (SEI), forms, which kinetically stabilizes the electrolyte. Similar considerations hold for the cathode interface, especially in case of high voltage electrodes, requiring an analogous Cathode Electrolyte Interphase (CEI).
In practice, the formation of these passivation layers can be achieved using sacrificial additives. However, in many cases the detailed breakdown mechanisms remain unclear, hampering a directed improvement of these additives. By means of Quantum Chemistry calculations, Diddens aims to rationalize redox stabilities observed by experimental collaborators, explicitly taking potential decomposition reactions (5; 6; 7) and polarization effects due to ions and solvent molecules in the intermolecular environment (8; 9) into account (figure 3). In this way quantitative agreement between experiment and computations can be achieved.
Publications:
1) Journal of The Electrochemical Society 2017, 164(11), DOI: 10.1149/2.0271711jes
3) Solid State Ionics 2020, 357, 115497, DOI: 10.1016/j.ssi.2020.115497
4) Physical Chemistry Chemical Physics 2018, 20(24), 16579-16591, DOI: 10.1039/C8CP01485J
5) Journal of Power Sources 2020, 461, 228159, DOI: 10.1016/j.jpowsour.2020.228159
6) ChemElectroChem 2020, 7(6), 1499-1508, DOI: 10.1002/celc.202000386
7) Applied Materials & Interfaces 2019, 11(18), 16605-16618, DOI: 10.1021/acsami.9b03359
8) Journal of Power Sources 2020, 478, 229047, DOI: 10.1016/j.jpowsour.2020.229047
9) Journal of Chemical Physics 2020, 152(17), 174701, DOI: 10.1063/5.0003098